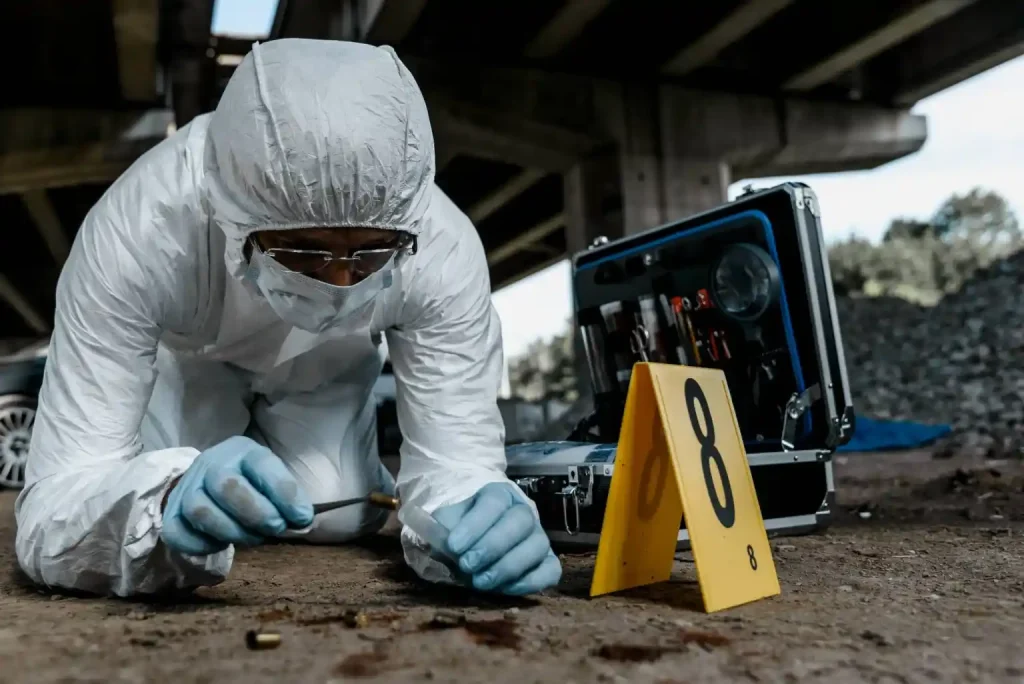
Depending on whom you speak with the term forensics can be a dirty word. It either conjures up images of flawed feature-comparison disciplines (hair-, bite-, or tool-mark comparisons for example) underlined by poor science or single source DNA which exonerates the falsely accused. PFAS forensics, when practiced well, more closely resembles DNA evidence for a criminal case and may play a critical role in ensuring those responsible for contamination pay for its remediation. The purpose of this article is to provide a gentle introduction to environmental forensics with an emphasis on PFAS. This is just one of several articles I have written on PFAS, it may help to refresh on PFAS terminology as well as PFAS analytic strategies. I am acting in my own capacity, my views are my own and do not represent my employer. Nothing here constitutes legal or professional advice.
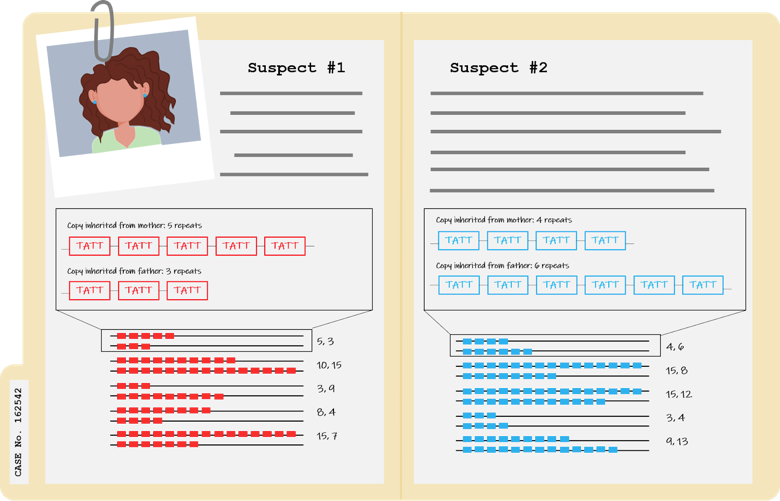
Introduction to PFAS Forensics
PFAS forensics is not a bunch of EPA investigators playing “good cop bad cop” with a chemical manufacturer or waste manager tied to a chair with a bright spotlight shining in their face. PFAS forensics is the subset of environmental forensics which deals with PFAS. Environmental forensics more generally is really the logical outgrowth and terminus of one branch in fate and contaminant transport. While fate and contaminant transport in general describes how chemicals move and transform throughout the environment, when this is reframed through the lens of forensics the emphasis shifts from more describing mobility and persistence to assist in assessing exposure more to source identification/attribution as well as developing legal along with scientific defensibility of conclusions. With billions on the line in multidistrict litigation you can bet the science will be heavily scrutinized.
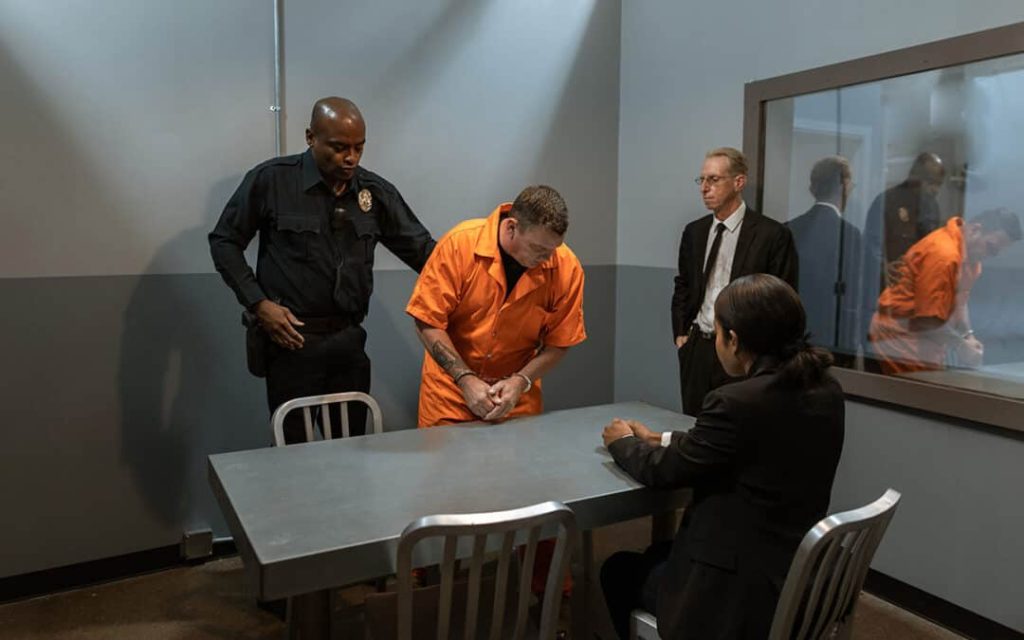
Basic PFAS and Environmental Forensics
Environmental forensics, and by extension PFAS forensics, typically has a few routine questions:
- Who is responsible for the PFAS?
- When and how was the PFAS released?
- In cases with more than one responsible party: How much has each party contributed?
- Were the releases progressive or sudden?
Environmental forensics uses several techniques to answer these questions to tease out information about how the contaminant (PFAS in this case) got to the place where it was detected. In many cases multiple releases of the same or related chemical are from the same site which may require contribution allocation for each identified release – this is especially important where more than one responsible party is identified. Beyond obvious applications in litigation, PFAS forensics could potentially lead to cost savings by better characterizing the sites and understanding related risks from them, better tailoring remediation or treatment, and assisting in insurance claims. In some cases, environmental forensics has also lead to completely new discoveries. For example, an unknown natural perchlorate source, unrelated to nitrate from the Atacama Desert or other known sources, was discovered using isotropic fingerprinting during a forensic investigation launched after routine perchlorate monitoring discovered contamination north of San Diego. While a PFAS forensic study may be solved using only one technique it is important to build multiple independent lines of evidence consisting of at least two distinct techniques to check your work. The independent evidence should obviously point to the same conclusion. Cases where one line of evidence is enough tend to include straightforward reported releases or dumping.
Common Non-Intrusive Environmental and PFAS Forensic Techniques
When performing document review, it’s critical to pay attention to chemicals which while may not pose health risks may be linked to the original health contaminant as an additive or may influence the fate and transport of the contaminant of concern. Site features such as underground storage tanks, https://www.epa.gov/ust ditches, loading docks, transformers/electrical components (especially for PCBs) are also important.
- Historical document review which can include historical documents (deeds, spill, or ownership records), environmental permits, operational or monitoring data, shipping manifests, as well as sales or invoices can be extremely helpful. These can also assist in recreating production processes if no records exist.
- Photographic review is very valuable. Systematic coverage of the US has occurred since the 1930s and changes overtime can be very instructive. The Aerial Photography Field Office of the USDA is particularly valuable and infrared photography can also assist in differentiating vegetation changes.
- Map review which can denote things such as waste or chemical storage, loading/unloading areas, warehouses etc… Sanborn maps (fire insurance maps) are particularly useful.
- Interviews with current and past employees, neighbors, or other relevant parties can provide clues to focusing the search or turn up undocumented knowledge.
- Market forensics started due to diffuse releases, for example in consumer products. Essentially sales and other relevant product information (eg formulation) is aggregated to estimate total loading. Market forensics has been successfully used on a local scale to determine fatty alcohol (detergent) contamination in Luray, Virginia (home of the caverns). I believe this to be most accurate for PFAS on a global scale as some emissions inventories have shown. In most instances on local scales it is closer to bite mark analysis (forensic odontology) than DNA analysis.
A whole review industry has sprung up from historical review in the form of EPA Phase 1 Environmental Site Assessments compliant with the All Appropriate Inquires Rule from 40 CRF 312. This can be considered defensive due diligence when performed prior to a real estate transaction.
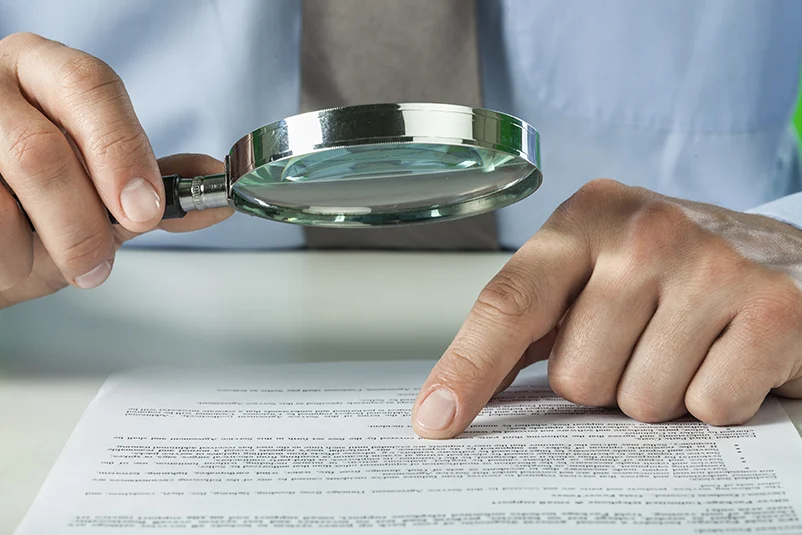
Advanced Environmental and PFAS Forensic Techniques
Non-intrusive techniques alone may be enough to end investigations into PFAS sources however, sometimes more advanced techniques are required. These advanced techniques should only be undertaken after integrating any existing knowledge body related to a specific site because advanced techniques are often costly.
Chemical Fingerprinting
Chemical fingerprinting is where specific sources are associated with characteristic distributions typically developed through comparison source samples or a priori knowledge developed using non-intrusive techniques. Chemical fingerprinting has successfully been used for PFAS source identification several times.
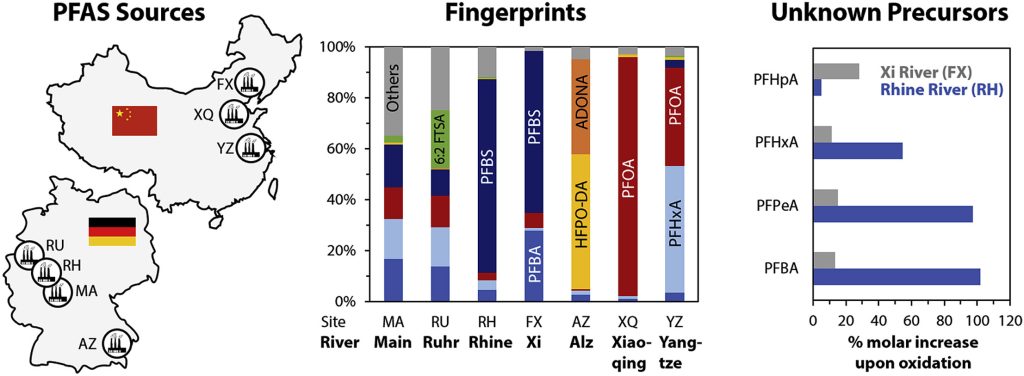
Isomer Analysis
Isomer analysis, isomers are compounds with the same formula but differing chemical structures. PFAS are generally produced through either electrochemical fluorination (ECF) or telomerization. ECF products contain approximately 70–80% linear structures and 20–30% branched isomers; telomerization yields relatively pure linear or isopropyl branched isomers. This technique also has been used several times for PFAS source identification.
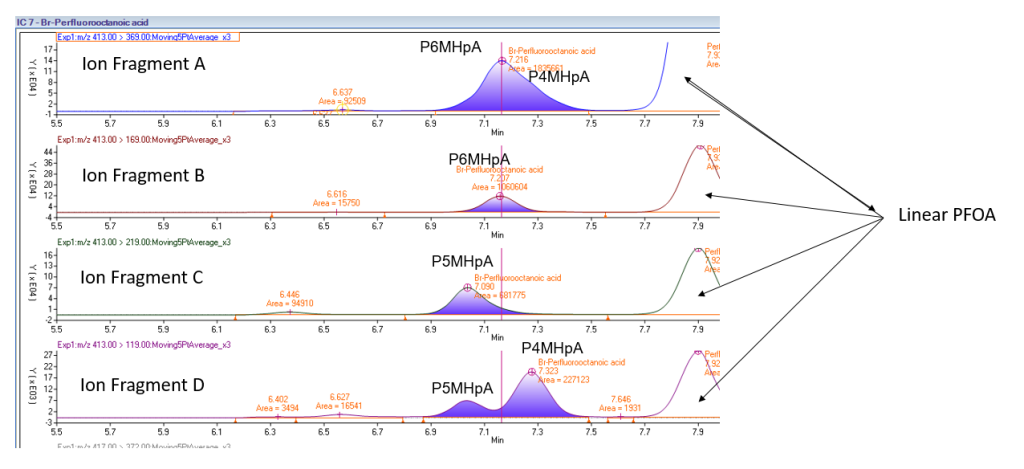
Chiral Analysis
Chiral analysis is a very unique subset of isomer analysis. The easiest way to think about chiral isomers, or enantiomers as they’re also known, is your right and left hand. Your hands are the same “isomer” except for an asymmetry so if you line your hands on top of each other palms down they do not match however, palms together they do. This is the same concept behind chirality. Manufactured compounds (such as PFAS) are racemic mixtures which means they contain the same or near equal enantiomer ratios. Since enzymes are always chiral, biotransformation is normally enantioselective. Chiral analysis can show if biotic uptake is due to precursors or direct source and has been used for this purpose. While I am not aware of any studies, this kind of analysis may also be able to differentiate weathering and precursor transformation for example between manufactured PFOA against PFOA degraded from fluorotelomer alcohols.
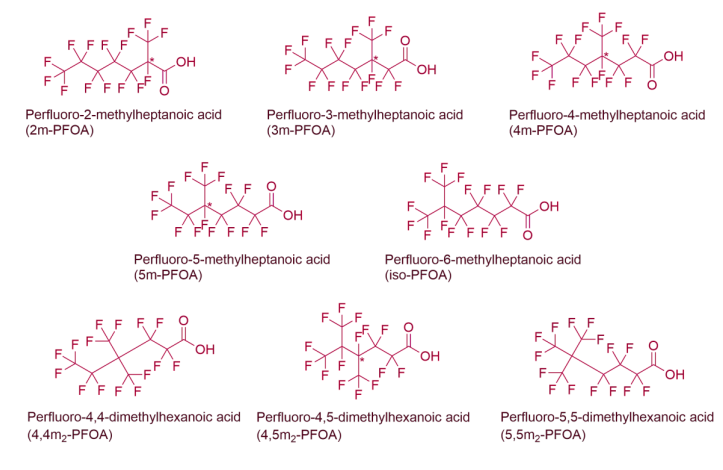
Isotropic Analysis
While isomers have the same chemical formula but distinct structures; isotopes are elements that have the same atomic number but different atomic mass. Isotopic Analysis means techniques measuring the mass of different stable isotopes, typically carbon, hydrogen, oxygen, nitrogen, and chlorine although there are many other possibilities. Unless something is ionized its protons must balance charge with electrons however, neutrons, which carry no charge, affect the weight allowing for this type of analysis. There are three main Isotopic Analyses which fall into this category: bulk, compound specific, and positional specific.
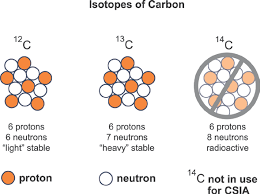
Bulk Stable Isotropic Analysis (BSIA)
Bulk Stable Isotropic Analysis is a specific type of isotropic analysis that measures the total concentrations of stable elements in a sample and can be correlated to the specific element in the releasing source. While I do not know any direct applications to PFAS yet, this technique has been used before to combat pharmaceutical counterfeiting.
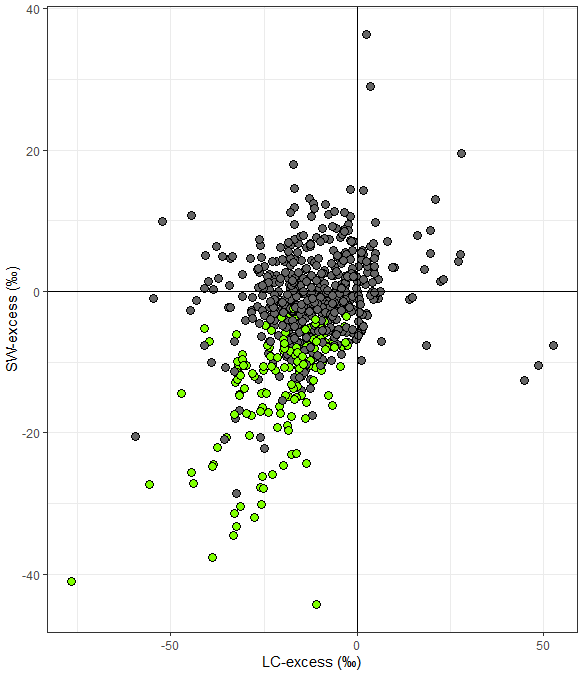
Compound specific isotopic analysis (CSIA)
Compound specific isotopic analysis (CSIA) is isotropic analysis for specific compounds (for example PFOA) within a sample and is generally much more powerful a method. This is in the process of being applied to PFAS, through Strategic Environmental Research and Development Program (SERDP) and Environmental Security Technology Certification Program (ESTCP) project ER24-4239.
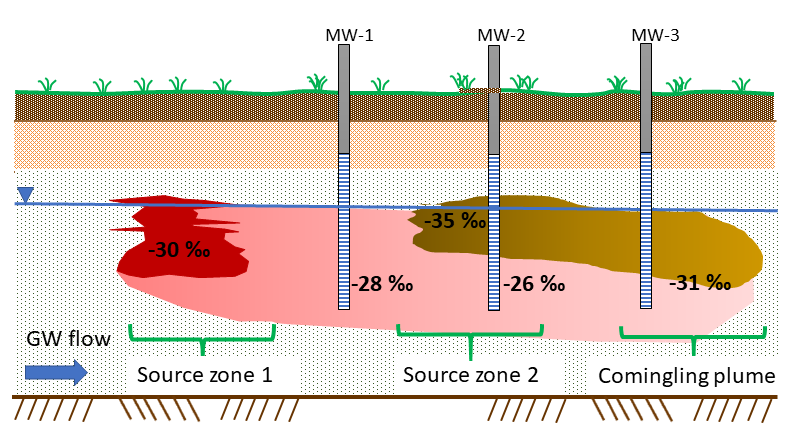
Position specific isotopic analysis (PSIA)
Position specific isotopic analysis (PSIA) provides stable isotope ratios at each carbon position where a C–F bond is present. This has been done with PFAS in the lab and theoretically is the most powerful isotopic analysis method available.
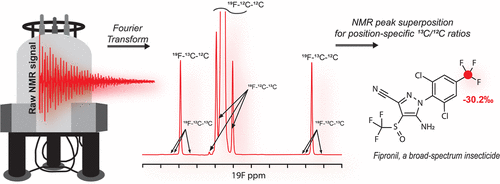
Advanced Statistical Techniques
Advanced statistical techniques such as principal component analysis can also assist in forensic studies. PCA is designed to reduce a dataset to its principal components by taking an original dataset and finding a new smaller set of variables that still describes the most variance in the original dataset – that is to say to take the chemical inputs and reduce it to source inputs. PCA relies on eigen values and eigen vectors to reduce the dimensionality of a dataset.
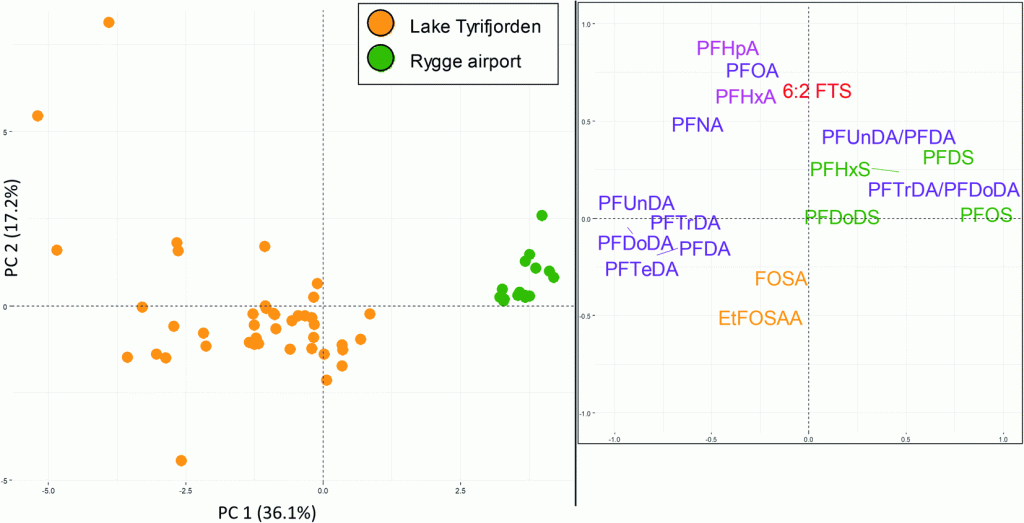
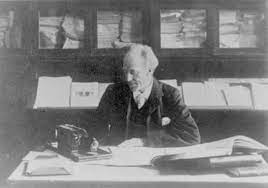
Dendrochronology
Dendrochronology, or dating tree rings, may provide inference on when PFAS releases first occurred, particularly for birch or spruce although I do not think this has been used in this way yet; most information revolves around phytoremediation. Dendrochronology may not yet meet the Daubert Criteria (some states still use the Frye Standard but all federal courts use Daubert) but there is some evidence for it.
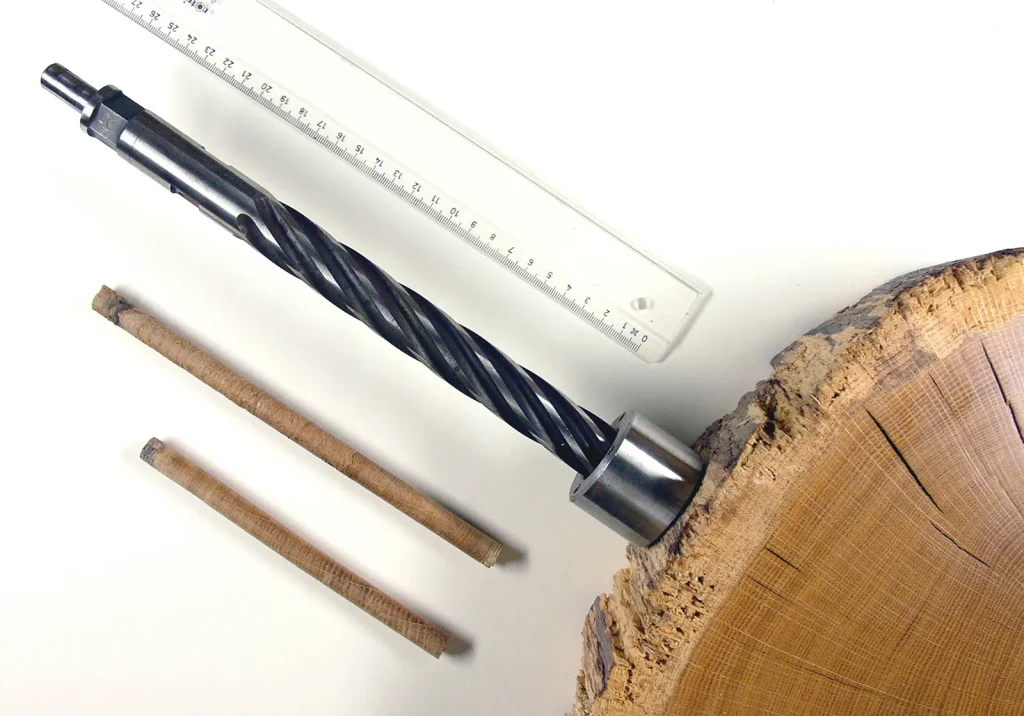
Some PFAS Forensics Considerations
PFAS forensics is a rapidly evolving science with many unknowns. However, it can draw many parallels to petroleum forensics which has a very deep body of knowledge around it because of the environmental liability associated with petroleum. For example, both are made of complex chemical mixtures enabling chemical fingerprinting for ready source identification. Despite a varied chemical composition, there are a few constants with PFAS. For example, Perfluoroalkyl acids (PFAAs) are strong acids that are anionic at environmentally-relevant pH. PFAAs are extremely persistent in the environment and do not degrade or transform under typical environmental conditions polyfluoroalkyl substances include compounds that have the potential to transform into PFAAs and are known as precursors. Carbon chain length and functional moiety control sorption and therefore transport. High sorption capacity implies slower environmental transport and vice versa.
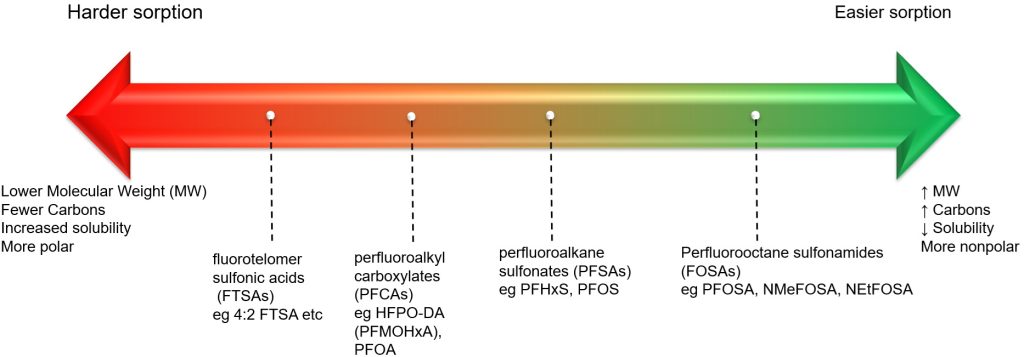
Some specifics that make PFAS forensics unique are:
- With no known natural formation there is a source responsible somewhere.
- Formulation can help establish dates. PFAS are entirely anthropogenic first starting in 1938 with several types not produced till much later. Presence of ether-ified PFAS, such as HFPO-DA (GenX Chemicals), for instance implies a 2000s source at the earliest.
- Broad industrial use means many sources exist and differentiation may be important.
- PFAS typically had industrial formulations to meet performance standards eg being able to extinguish a fire in a set time and not chemical composition standards so the chemical composition varies through time. In other words PFAS formulation depends on production year. Commercial formulation and usage profiles can identify sources.
- Individual PFAS measured are only a fraction of total PFAS.
- Lowered measured concentrations lead to increased uncertainty.
- Due to long range transport capabilities, PFAS are ubiquitous global contaminants. For instance, rainwater has been found to exceed the new PFAS drinking water regulation.
- Transport properties can additional sources. For example, if PFOA is ahead of PFHxA it can indicated separate releases since the longer chained PFAS has lower transport capabilities.
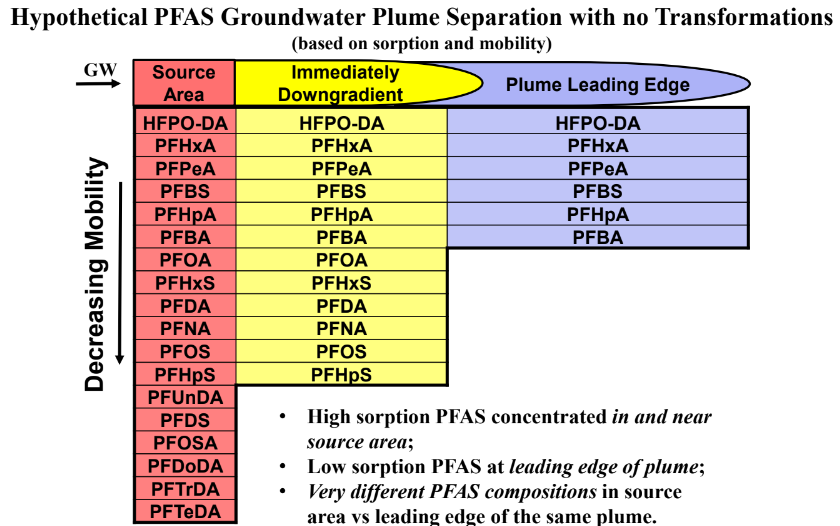
- PFAS are generally resistant to weathering and transformation (hence the term “forever chemicals”) however, precursors may occur. For example fluorotelomer alcohols may transform into perfluoroalkyl carboxylic acids.
- Ratios of precursors (such as FTOHs) to terminal end products (PFAAs) should increase (ie fewer FTOHs and more PFAAs) with time and distance from sources. There should be a precursor gradient in other words.
- PFAS tend to accumulate at interfaces for example between bulk liquids and L- or D-NAPLs, soil/water, soil/water and the atmosphere and so forth. In fact, this tendency has lead to foam fractionation as a potential treatment method.
- PFAS tend to accumulate in muscle as opposed to adipose (fat) tissue unlike most contaminants.
- Linear compared to branched PFAS should increase with distance from point source because most Br-PFAS have a higher water solubility and faster biological elimination from organisms which also results in faster biotransformation closer to the source.
- Due to biotransformation, specific environmental PFAS concentrations should not be used to predict biotic uptake (take total organofluorine into account).
- Low water concentrations compared to biota (hence implied high bioaccumulation factors) can indicate that water is not the only source.
- Age-dating may be possible using specific diagnostic ratios.
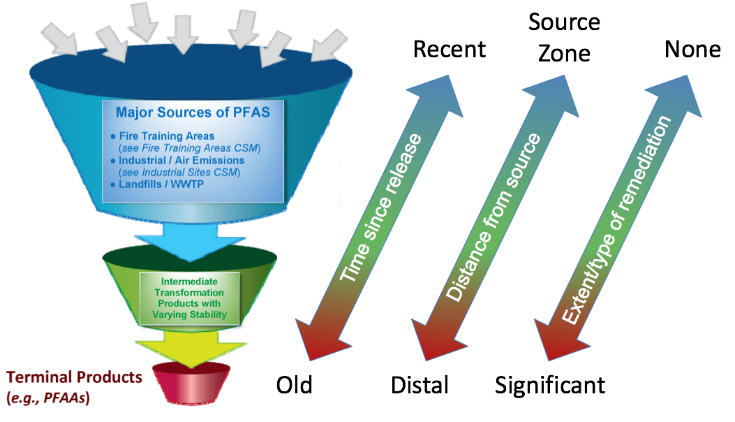
Conclusion and Key Take Aways
Practically, it is impossible to remediate global PFAS contamination however, managing PFAS hotspots through forensic investigations may significantly reduce global contamination’s extent. There are many parallels which can be drawn between petroleum forensic investigations and PFAS as well as many well-developed techniques to assist in source identification however, it is still a rapidly evolving field. The goal of PFAS forensics, and really environmental forensics, is ultimately to develop legal as well as scientific defensible conclusions related to source attribution. To that end, multiple independent lines of evidence should be used to validate and strengthen conclusions. If you learned something or enjoyed this article (I know I separated those but for me they tend to be correlated!) take a look at some other articles I have written on PFAS.